R&D
What is Terpene Resins?
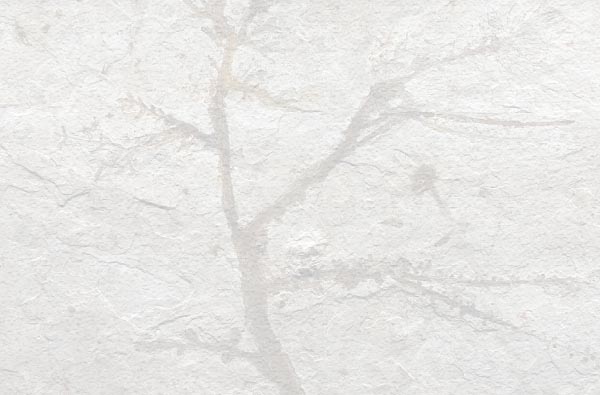
R&D
Pale amber, transparent, thermoplastic polyterpene hydrocarbon resins, of the type formula (C10H16)n, have been produced and sold on a commerical scale since 1938. These resins are characterized by ring-and-ball softening points (S.P.) ranging up to about 135°C. They are soluble in a great variety of organic solvents, including hydrocarbon solvents and are of good color stability. They are used, in conjunction with other materials, in the formulation of a wide variety of end products. including adhesives, adhesive tapes, rubber goods, and coating compositions. Depending on the nature of the end use, the terpene resins are supplied either in solid form or in solution in hydrocarbon solvents; the solid forms are made in a wide range of softening points or molecular weights.
These polyterpene resins are derived primarily from the catalytic polymerization, in solution, of the bicyclic monoterpene pinene, C10H16, principally the β-isomer (nopinene), and may be regarded as essentially polymers from β-Pinene. The pinene in turn is derived from gum and sulfate Turpentines, from both of which it is recovered by fractional distillation.
This article is concerned only with these hydrocarbon resins, and does not cover other terpene-derived resinous substances, such as Rosins (q.v.) and the Terpene-Phenol Resins. The latter are produced by reacting terpene hydrocarbons or alcohols with phenol in the presence of acid catalysts, followed by catalytic resinification of the resulting substituted phenol with a reactive substance such as formaldehyde. Such Terpene Modified Phenolic Resins exhibit among other properties increased solubility in drying oils. They are useful in adhesives and in various types of coatings, including particularly wax emulsions, varnishes, paints, and heat-setting printing inks. Certain resins are also antioxidants for rubber.
The commerically available Polyterpene Resins are produced with a variety of softening points (measured by the A.S.T.M ring-and-ball method E28-51T), ranging from 10~135 °C, corresponding to a range from viscous liquid to hard, brittle solid polymers at ordinary temperatures. The average molecular weights of these polymers increase as the softening points increase. A molecular weight of about 1200~1250, measured cryoscopically in benzene, is characteristic for a polymer of 125~135°C softening point. In common with polymers generally, the Polyterpenses are mixtures of polymers of various molecular weights and chain lengths. A characteristic molecular-weight distribution obtained by fractionation for a commercial 135°C softening point polyterpene resin is shown in Table 1. The fractionation was accomplished by partial precipitation from amyl alcohol, the general procedure involving solution of the resin in hot amyl alcohol, followed by cooling, decanting the amyl alcohol solution from the precipitated higher-molecular-weight polymers, and steam distillation of the decanted solution to remove the alcohol. Fraction 1-0 were so obtained, increasing amounts of amyl alcohol being required for the successive fractions because of the decreased solubility associated with increased molecular weight.
Fraction | Weight % of original resin | Molecular weight (cryoscopic in benzene) |
1 | 12.8 | 750 |
2 | 16.9 | 830 |
3 | 11.5 | 910 |
4 | 12.8 | 1150 |
5 | 12.9 | 1620 |
6 | 16.9 | 1910 |
7 | 16.2 | 3540 |
These polymers are typically thermoplastic, and merely soften or harden as they are beated or cooled, no irreversible change occurring as long as the heating temperature is kept below that at which pyrolytic reactions set in. In common with other amorphous hydrocarbon resins, these polymers show no sharply defined liquefaction or solidification temperatures, and their softening points are therefore measured by arbitrary standard test methods.
The polymers are slightly less dense than water, resulting in a relatively high bulking value compared to other polymeric materials. Values for the specific gravity of these resins range between about 0.94~1.00, depending on the softening point or molecular weight, the exact composition of the monomeric terpene mixture polymerized, and the method of production.
The resins exhibit in , r compatibility with, a wide range of materials. Complete miscibility exists with liquid paraffinic, naphthenic, and aromatic hydrocarbons, chlorinated hydrocarbons, higher alcohols, esters, and drying oils. Compatibility is exhibited with Rosin, Ester Gum, waxes, including paraffin wax, polysibutylenes, petroleum residues and pitches, mineral oils, and certain types of petroleum hydrocarbon polymers. Under appropriate conditions, compatibility is exhibited with rubber and modified rubbers, including synthetic rubbers such as GR-S. Compatibility with cellulose and esters, is, however, quite limited.
Inasmuch as these resins re entirely hydrocarbon in nature they are moisture resistant and possess good dielectric properties.
As essentially pinene polymers, principally polymers from β-Pinene, the terpene resins exhibit properties typical of polymeric hydrocarbons including chemical inertness. They are inert to dilute mineral acids, alkalies, and salt solutions, and are characterized by acid numbers and saponification numbers less than four and approaching zero. Similarly they are resistant to heat over a wide range of temperatures, varying only in fluidity with temperature.
The detailed chemical structure of the terpene resins cannot be regarded as satisfactorily elucidated. Published data in the chemical literature and patented art have indicated these polymers to be susceptible to both halogenation and catalytic hydrogenation, and to reaction with ozone to give polymeric ozonides. For example, the reported weight of hydrogen absorbed by a catalytic polymer made from β-Pinene was 1.2%. This is equivalent to 0.82 mole of hydrogen per C10H16 recurring unit in the polymer chain. Based in part on these considerations, and in part on physical properties, Roberts and Day speculated that the catalytic polymerization of β-Pinene involved more than a simple chain addition reaction of monomer molecules and that isomerization occurred during the polymerization to give a polymer comprising a chain of recurring monocyclic C10H16 terpene units each containing a carbon double bond. Powers has also suggested the recurring unit of polymers pinene to be monocyclic and unsaturated, proposing a formula different in detail and in point of linkage.
More recent studies of these polymers by infrared spectrophotometric techniques have indicated that the polymers contain notably less unsaturation that would be required for a chain of recurring monocyclic terpene units but more than would be the case for a simple addition polymerization of β-Pinene molecules yielding a poly molecule containing only a single terminal double bond. Part, but not all of the unsaturation observed by infrared examination appears to represent terminal double bonds in the poly molecules inasmuch as it decreases with increasing molecular weight.
These observations imply that the pinene polymerization is, as postulated by Roberts and Day, more complex than simple addition, but that the final recurring unit structure is not solely monocyclic. Pending further elucidation it seems preferable toavoid the term poly-β-Pinene, insofar as the latter may imply simple addition polymerization.
Basically, terpene hydrocarbon resins are produced by the controlled treatment of the monomer in solution in a hydrocarbon diluent with a catalyst of the Friedel-Crafts type, such as anhydrous aluminum chloride. The polymerization reaction is highly exothermic, and provision is made for adequate agitation and heat exchange. On the completion of the reaction the mixture with steam at reduced pressures to remove solvent, any unreacted terpenes, and also any steam-volatile low-terpene polymers. The residual nonvolatile molten resin may then be poured into drums to cool, or used for the preparation of terpene resin solutions.
Of the two naturally occurring isomers of pinene, the β-form is greatly superior to the α-isomer in catalytic polymerization, with respect to polymer yield and polymer molecular weight or softening point and it was this finding which laid the basis for the β-Pinene occur in Gum Turpentine and in many crude sulfate Turpentine. In the case of gum spirits of Turpentine from longleaf pine, a typical composition is 63% α-Pinene, 33% β-Pinene, and 4% higher-boiling constituents. Moderate variations in these percentages occur with variation in the source, but the β-Pinene content is seldom below 29%. In contrast, sulfate turpentines may vary very widely in composition, depending on the geographical area source, and processing techniques involved. Usually the pinenes are accompanied by smaller quantities of higher-boiling monocyclic terpenes, particularly Dipentene (also called Limonene).
For appropriate use as charge material for polymerization to terpene resins, it is advantageous to separate by fractionation the bulk of the α-Pinene from the original Turpentine, and to prepare a relatively concentrated fraction of β-Pinene. Such processing not only yields highly concentrated β-Pinene of particular usefulness for making terpene resins, but also gives highly concentrated α-Pinene fractions which are themselves useful in other chemical processes, particularly the manufacture of synthetic camphor.
The boiling points of the isomeric pinenes, and also of Dipentene, are sufficiently close that relatively efficient fractionation is required for this purpose. These boiling points at atmospheric pressure are: α-Pinene 156°C, β-Pinene 165°C, and Dipentene 176.5°C.
Depending on the efficiency of fractionation, commerical β-Pinene will usually contain, as the principal other consituents, α-Pinene and Dipentene. Inasmuch as these are all C10H16 terpene isomers(CAS 31393-98-3 | CAS 34363-01-4 | CAS 25766-18-1 | CAS 70750-57-1 | CAS 25719-60-2 | CAS 9003-74-1), the empirical formula of the final polymer, (C10H16)n is not affected.
For effecting the polymerization reaction, the β-Pinene fraction is diluted with a refined hydrocarbon solvent, such as a naphtha cut. This dilution prevents the viscosity of the reaction mixture from becoming excessive as the polymerization reaction proceeds, thus facilitating agitation, circulation, heat exchange, and final catalyst removal. In practice, dilution of the pinene to a concentration between about 40~50% is satisfactory.
The conditions during polymerization are very closely controlled. In batch reaction it is necessary to add the Friedel-Crafts catalyst carefully and gradually because of the vigorously exothermic nature of the reaction. By careful control and instrumentation, temperatures are maintained within a few tenths of a degree, the reaction heat being removed by heat exchange with cold water or brine. Temperatures of polymerization are moderate, practical operations generally being within the range 20~50°C.
Usually, after heat evolution from the reactions has subsided, an additional contacting period with the catalyst is provided to insure essentially complete conversion of the monomers to polymer.
Before recovery of the resin itself, complete removal of the halide catalyst is necessary. This may be effected by successive washing with water and dilute aqueous alkali, followed by separation of the aqueous material from the hydrocarbon phase. Removal of the catalyst changes the reaction mixture from its original dark red color to a pale yellow. Special subsequent treating steps are used to remove final traces of halogen.
The catalyst-free, neutral resin solution is then charged to batch distillation kettles. At elevated temperatures, volatile oils are distilled out, final operating conditions of steam and vacuum being used to remove any more difficultly volatile, heavy, polymeric oils produced in the reaction. The recovered diluent naphtha may be recycled in subsequent charges to the polymerizer.
At the elevated stillpot temperatures (above 200°C) used in the finishing step, the final resin, free of diluent, unreacted terpenes, and steam-volatile heavy oils, is present as a viscous, pale amber liquid ready for transfer. Depending on the use to which the resin is to be put, the molten resin may be poured shipment, or it may be transferred to a separate vessel to make terpene resin solution. The resin solutions normally contain 45~65% solids in mineral spirits.
The different grades of commerical terpene resin, sold under the trade name Piecolyte, are produced by appropriate choice of conditions in the manufacturing step. These grades range from 10°C S.P. to 135°C S.P., the lower-softening point products being viscous liquids at ordinary temperature. For shipment, these soft or plastic grades are poured into heavy drums of the open-head type, the soiled grades are poured into light-gage drums.
For certain specialty uses where a water-white terpene resin is required, the regular, pale amber product is catalytically hydrogenated in solution, to reduce the unsaturation and color, and to permit the recovery, on redistillation of the solvent, of an essentially colorless resin product.
Economic Aspects. The terpene resins have take their place in a number of specialty uses industrially, for many of which no other resin has been found to possess equally satisfactory characteristics. Although their production is limited in comparison with many other polymeric products, such as phenolics and molding resins, such production has increased substantially since there initial manufacture in the late 1930’s by the Pennsylvania Industrial Chemical Corporation (PICO).
Their manufacture from raw materials derived from Turpentine represents a chemical utilization of a naturally occurring product, and is to that extent helpful in stabilizing Turpentine operations and marketing. They provide a use for β-Pinene , whereas other chemical uses of terpene hydrocarbons from Turpentine are largely based on α-Pinene as a raw material.
Inasmuch as β-Pinene fractions are recoverable from both gum spirits of Turpentine and from many crude sulfate Turpentines, the production of terpene resins is connected with both the naval stores and pulping industries, and the magnitude of production is controlled in part by the magnitude of gum spirits and pulping operations.
In July 1954, the selling price of the resins ranged between $0.185 and $ 0.200 per pound depending on quantity and on the softening-point grade.
The field of usefulness for terpene resins is based especially on the combination of solubility, light color, stability, and chemical inertness, as well as their lack of toxicity. Particularly important to the use pattern for terpene resins are the very definite and desirable adhesive properties imparted to formulations involving natural or synthetic rubbers. They are usually used in conjunction with other materials in various industrial formulations.
The wide application of the resins in adhesives and in the reparation of adhesive tapes includes their use in rubber cements, friction tapes, and with polyisobutylene. Another wide field of application is in rubber goods, including toys, hose and mechanical rubber goods. The resins are also useful in paints and varnishes, concrete curing, chewing gum, paper coatings, and leather goods, as well as in laminating and polish waxes, and in inks. The compatibility between terpene resins and natural and synthetic rubbers is of particular importance, since a number of theses uses are based on formulations involving such mixtures.
-above article is written by By H. Panda, Pages 208~214, Handbook on Oleoresin and Pine Chemicals.