Home > Sustainability > Terpene Compounds
Unveiling Carbon Footprints through the Lens of Biochemistry and Terpenes
Carbon Footprints and Carbon Reduction Actions
Carbon footprint refers to the total amount of greenhouse gases (GHG), primarily carbon dioxide (CO2), methane (CH4), and nitrous oxide (N2O). According to standards such as ISO-14044, ISO-14067, and PAS 2050, all greenhouse gas emissions generated by a product at each stage of its lifecycle are accounted for. Fossil energy emissions data from the China Carbon Accounting Database for 2023 show that coal ranks first as the primary source, followed by petroleum products. Analyzing emissions by industry, the main contributors are the electricity, heat, gas, and water infrastructure sectors, followed by the metal products manufacturing industry and the transportation and warehousing industry.
Within most industries, this process involves sourcing raw materials, manufacturing the product, transporting the final product, and the consumer’s use and disposal of it. The EPA’s monitoring and assessment of commercial activities in North America reveal that emissions from different stages of the value chain (not categorized by industry) are distributed as follows: transportation of goods (35%), electricity usage (30%), manufacturing processes (16%), residential and commercial sectors (12%), and miscellaneous sources (7%). Decreasing carbon emissions from these activities is crucial for meeting carbon reduction goals.
Raw materials suppliers of pine-based modified polymer resin typically rely on cradle-to-gate calculations to assess carbon emissions in the industry, tracking from raw material acquisition to polymer production completion. The ink manufacturer Avient presents strategies for lowering the carbon footprint through the three stages of the polymer value chain.
- Raw Material Suppliers. Suppliers that source bio-based and recyclable raw materials generally have a greater impact on reducing carbon emissions. Bio-based polymers are typically derived from various renewable resources, such as corn and straw. Polymers containing bio-based components, especially those using raw materials extracted from plants, consume carbon dioxide and are often considered to have negative carbon emissions. Hence, it is advantageous to encourage polymer manufacturers and raw material suppliers to maintain consistent sustainability commitments in order to decrease carbon emissions.
- Polymer Manufacturers. Polymer manufacturers can reduce their carbon footprint by using efficient production equipment and renewable energy, as well as recycling waste generated during the production process.
- Brand Owners or OEMs. Brand owners or OEMs can reduce their carbon footprint by making environmentally friendly changes in various ways, such as in the production of final products, transportation of final products to distributors, and customer disposal or use of the products.
In the fragrance and flavor industry, the synthesis of vanillin is primarily done using fossil fuel routes from eugenol or guaiacol derived from crude oil. The Norwegian company Borregaard is dedicated to producing a natural equivalent of vanillin using lignin extracted from Norway spruce. Compared to other petrochemical companies, Borregaard has achieved a 90% reduction in CO2 emissions in the value chain of lignin-based vanillin production. The Japanese petrochemical company Eneos is increasing its focus on hydrogen-based energy management and investing in the development of bioethanol technology. Eneos uses xylose yeast for saccharification and fermentation to produce cellulosic bioethanol, with plans to reach commercial scale by 2027. BASF has introduced zero carbon footprint neopentyl glycol (NPG) and propionic acid (PA) products, aiming to achieve CO2 reduction targets in powder coating applications and the feed, food, agriculture, and fragrance industries.
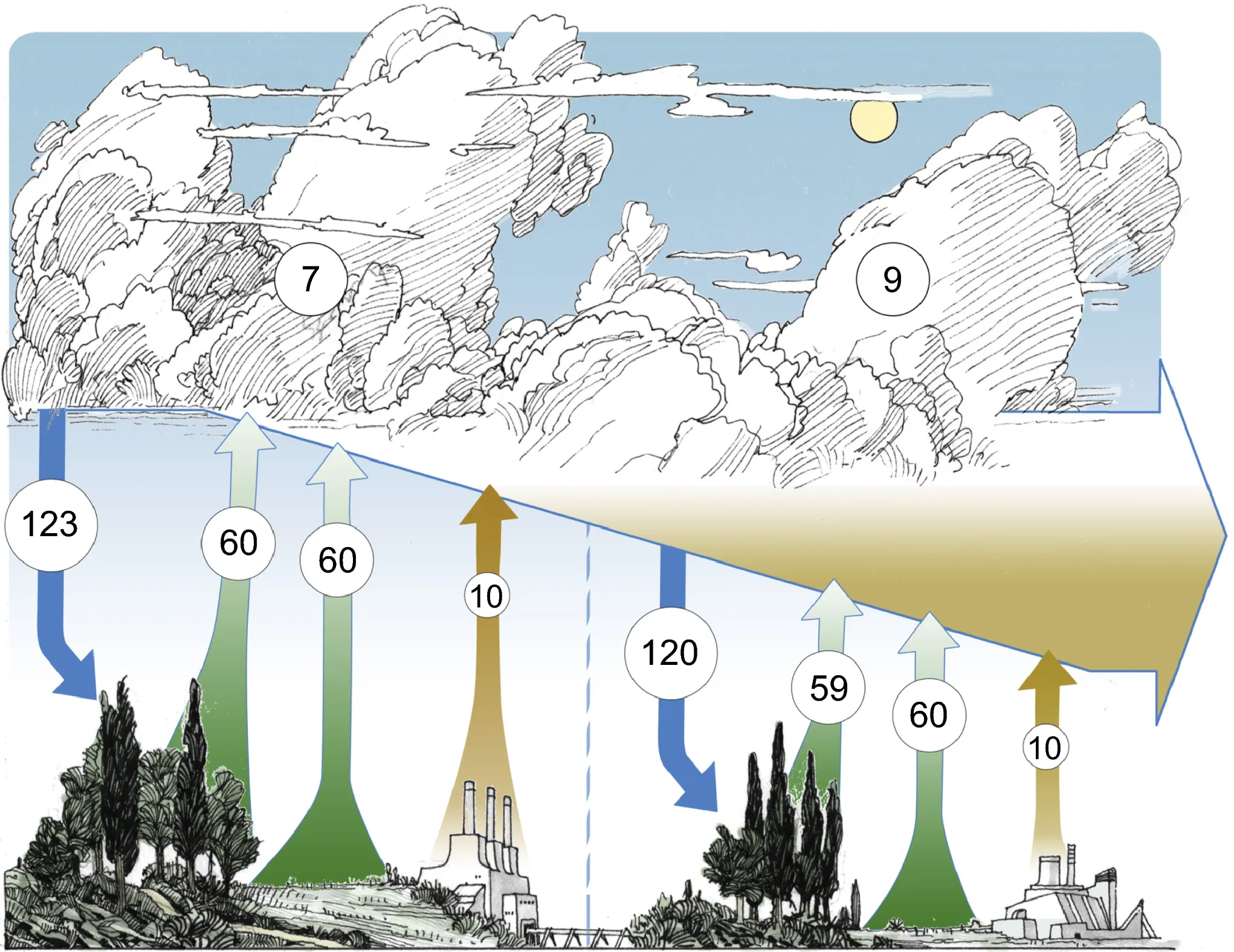
Figure 1. A simplified schematic of the interplay between the climate system and carbon (C) fluxes in vegetation. [Source: © Mirindi Eric Dusenge al., Plant carbon metabolism and climate change: elevated CO2 and temperature impacts on photosynthesis, photorespiration and respiration, New Phytologist 211: 32-49.]
![figure-sustainability-_Diagram_representing_the_juxtaposition_-in_two_types_of_cells-_of_the_C4_and_C3_cycles_in_plants_with_C4_type_metabolism. Figure 2. Diagram representing the juxtaposition -in two types of cells- of the C4 (mesophyll cells) and C3 (sheath cells) cycles in plants with C4 type metabolism (left). The C4 cycle enables CO2 to be concentrated in the vicinity of the RubisCO, thus promoting its carboxylase activity (Schéma Roger Prat, in Morot-Gaudry, Dunod, 2009). PGA, phosphoglyceric acid; RuBP, ribulose bisphosphate; PEP, phosphoenolpyruvate; HCO3-, bicarbonate. Right: Anatomy of a corn leaf section, a C4 plant. In light blue: chloroplasts of the mesophyll cells (C4); in blue-violet: chloroplasts of the perivascular sheath (C3). In the center, the cells of the conducting vessels. [Source: © Photo Frédéric Dubois, Université de Picardie]](https://foreverest.net/uploads/2024/06/figure-sustainability-_Diagram_representing_the_juxtaposition_-in_two_types_of_cells-_of_the_C4_and_C3_cycles_in_plants_with_C4_type_metabolism_7549.webp)
Figure 2. Diagram representing the juxtaposition -in two types of cells- of the C4 (mesophyll cells) and C3 (sheath cells) cycles in plants with C4 type metabolism (left). The C4 cycle enables CO2 to be concentrated in the vicinity of the RubisCO, thus promoting its carboxylase activity (Schéma Roger Prat, in Morot-Gaudry, Dunod, 2009). PGA, phosphoglyceric acid; RuBP, ribulose bisphosphate; PEP, phosphoenolpyruvate; HCO3-, bicarbonate. Right: Anatomy of a corn leaf section, a C4 plant. In light blue: chloroplasts of the mesophyll cells (C4); in blue-violet: chloroplasts of the perivascular sheath (C3). In the center, the cells of the conducting vessels. [Source: © The path of carbon in photosynthesis, Encyclopedia of the Environment]
Photosynthesis
Photosynthesis, biochemistry
Climate change affects the chemical and biophysical properties of proteins and DNA in organisms. Stress responses like coral bleaching and sex determination in sea turtles are activated in biological cell signaling pathways due to climate change [1]. The more carbon emissions accumulate from human activities, the higher the rate of photosynthesis in plants becomes as CO2 levels increase. According to relevant research (Figure 1), terrestrial plants absorb about 123 Gt of carbon from the atmosphere annually through photosynthesis and return about 60 Gt of carbon to the atmosphere through photorespiration [2].
Although plant leaves have an optimal temperature for CO2 absorption and can be acclimated according to environmental conditions, C3 plants need to close their stomata to prevent water loss when the ambient temperature rises. This closure reduces the CO2 concentration in the leaves, leading to a halt in the dark reactions. When the dark reactions cannot promptly consume the excess ATP, the likelihood of singlet oxygen formation increases, resulting in photoinhibition.
C4 plants, which grow in warmer regions, undergo less photorespiration (Figure 2). For example, maize has a biochemical mechanism that physically separates CO2 fixation from Rubisco. Its leaves have mesophyll cells surrounding the outermost vascular tissues, where oxaloacetate is converted into another C4 compound, malate. The malate is then transported to the bundle sheath cells surrounding the central vascular bundle. In these cells, the C4 compound undergoes enzymatic decarboxylation, creating a high concentration of CO2 around Rubisco, thereby promoting its carboxylation activity and subsequently regenerating phosphoenolpyruvate [3]. This mechanism in maize consumes more ATP to physically separate the biochemical processes of CO2 capture and utilization in Rubisco. C4 plants typically undergo less photorespiration and more efficiently fix atmospheric CO2.
In contrast, C3 crops such as wheat and soybeans exhibit strong temperature dependency in the oxidation and carboxylation activities of Rubisco during photorespiration. According to relevant research and modeling, photorespiration reduces the yields of soybeans and wheat in the United States by 36% and 20%, respectively. Additionally, yield reductions are more severe in warmer, lower latitude regions compared to higher latitude regions [4].
Photosynthesis and terpenes
Terpene compounds are a common type of biogenic volatile organic compounds (BVOCs) and are among the primary secondary metabolites synthesized by plant organisms. According to relevant models [5], the current global annual flux of BVOCs (excluding methane) is approximately 1000 Tg C, with isoprene accounting for 44%, monoterpenes for 11%, other reactive VOCs for 22.5%, and other VOCs for 22.5%. Based on relevant modeling, the emissions of 41 monoterpenes and 32 sesquiterpenes together account for approximately 15% and 3% of the global total BVOCs emissions [6], respectively. The synthesis and release of monoterpene compounds have a direct impact on ozone and the global carbon budget.
Table 1. Global annual total emissions simulated for the year 2000 using MEGAN2.1 algorithms in CLM4. [Source © A. B. Guenther al., The Model of Emissions of Gases and Aerosols from Nature version 2.1 (MEGAN2.1): an extended and updated framework for modeling biogenic emissions, Geosientific Model Development, 2012] | ||
Compound Class | Compounds | Emissions (Tgyr-1) |
α-Pinene | α-Pinene | 66.1 |
Acetone | Acetone | 43.7 |
Stress VOC | Ethene | 26.9 |
Bidrectional VOC | Ethanol | 20.7 |
Bidrectional VOC | Acetaldehyde | 20.7 |
t-β-Ocimene | t-β-Ocimene | 19.4 |
β-Pinene | β-Pinene | 18.9 |
Other VOC | Propene | 15.8 |
Other monoterpenes | Additional 31 monoterpenes | 14.9 |
Limonene | Limonene | 11.4 |
THE ENDLESS POSSIBILITIES WITH RENEWABLE RESOURCES
Terpene compounds, Polyterpene resins and Carbon footprint
Why is it said that pine chemical industry plays a positive role in carbon footprint? In the industrial chain in Europe and America, the pine chemical industry is based on the by-products of pulp production. The softwood pulp chips from sawmills are used for pulp production and to separate crude tall oil substances. The remaining residues are transported by BLS to chemical plants for acidification processes, producing downstream derivatives including crude sulfate turpentine (CST), tall oil fatty acids (TOFA), tall oil rosin (TOR), and tall oil pitch (TOP). According to statistical analysis by Franklin Associates [7], the carbon footprint emissions contributions within the aforementioned production lifecycle are ranked from high to low as pulping, forestry cultivation, distillation, or acidulation (Table 2.). Due to differences in fuel usage strategies between American and European companies, European companies use a higher proportion of biomass and other renewable materials. This results in American companies emitting nearly 11 times more carbon in the distillation stage compared to European companies. Through statistical comparison, American companies emit 1466 Kg CO2 per ton of CTO product produced, while European companies emit 740 Kg CO2. Since the CTO industrial chain is mainly established in Europe and America, the weighted average carbon footprint of global CTO products is approximately 1171 Kg CO2 eq/Tone.
Further carbon footprint analysis of energy consumption within the aforementioned lifecycle conducted by Franklin Associates concluded: Regardless of whether it’s American or European companies, the use of biomass fuel and other renewable fuels accounts for 81% to 86% of the total energy consumption in the CTO production lifecycle (Figure 3.). This result, compared to the lifecycle of the petrochemical route, undoubtedly plays a positive role in carbon reduction.
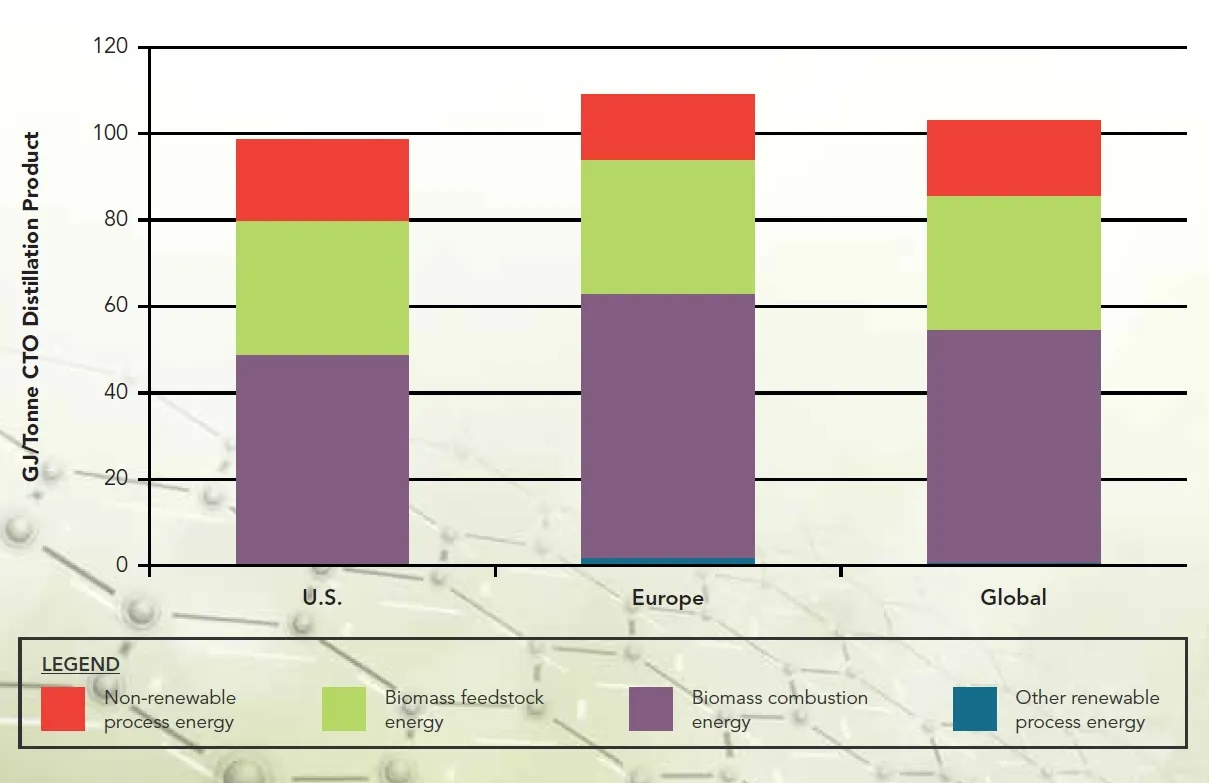
Figure 3. Breakdown of Baseline Total Energy Footprint Results by Geographic Region [Source: © Figure ES-4, Greenhouse Gas and Energy Life Cycle Assessment of Pine Chemicals Derived from Crude Tall Oil and Their Substitutes, Pine Chemistry Panel – The American Chemistry Council]
Comparing the CTO industry chain in Europe and America, The pine chemical industry presents another characteristic in China. Currently, it mainly relies on the manual collection of oleoresin. After the oleoresin collected by forest farmers, it is purchased and then enters the factory for the next production activities. This traditional resin collection behavior is concentrated in forest areas in Fujian, Guangxi, Guangdong, Yunnan, and other regions of China, with Masson pine, Yunnan pine, and Simao pine as the main tree species, among which Masson pine forests have the largest area [8]. Chinese forest farmers have been using the downward resin collection method since the 1960s [9]. According to the standard “LY/T1694-2007 Technical Regulations for Resin Collection,” issued by the China National Forestry Bureau, (Southern) forest farmers go to the mountains from April to November to collect resin using resin collection tools. Manual labor is primarily used during the resin collection process, and the degree of mechanization is low, keeping the carbon emissions at a relatively low level during this stage.
Carbon footprint calculations integrated into the lifecycle of CTO derivatives production can provide direct reference for China’s pine chemical industry. Although there is currently no comprehensive carbon footprint report for China’s pine chemical industry, it can be foreseen that in the cradle-to-gate (CTG) lifecycle, increasing the use of bio-based fuels and other renewable fuels, and widely applying clean energy in resin collection, transportation, and preparation processes, can significantly reduce carbon emissions.
The American resin company Kraton has received the EcoVadis Platinum rating for multiple consecutive years. Peter Migchels, the Director of Adhesive Marketing, once explained in an interview with the China Adhesives and Tape Industry Association (CATIA): “The bioeconomy relies on bio-based materials and products. When a material is wholly or partially derived from biomass, it is considered a bio-based material. Biomass refers to any material derived from living organisms, excluding substances buried in geological formations and/or fossils. [10]” He explained the concept of “industrial symbiosis”: “The process where by-products from one industry or process become raw materials for another industry. This concept enables materials to be used in a more resource-efficient and sustainable manner, serving as a key part of the circular economy.
Total | 1,466 | 100% | 740 | 100% | 1,171 | 100% |
U.S | Europe | Global | ||||
Cultivation through pulpwood to mill | 300 | 20.5% | 148 | 20.0% | 238 | 20.4% |
Kraft pulping (BLS as co-product) | 559 | 38.1% | 451 | 60.9% | 515 | 44.0% |
BLS transpotation | 16.2 | 1.10% | 2.55 | 0.34% | 10.7 | 0.91% |
Acidulation | 173 | 11.8% | 76.4 | 10.3% | 134 | 11.4% |
CTO Transportation | 15.4 | 1.05% | 25.6 | 3.46% | 19.5 | 1.67% |
Distillation | 402 | 27.4% | 36.7 | 4.96% | 254 | 21.7% |
Terpene compounds, Composite materials and Bio-based solvents
Structural diversity
Terpenes are a class of terpene compounds found abundantly in plants such as pine trees, eucalyptus, and citrus. They are widely distributed in the leaves, flowers, stems, and roots of these plants. Terpenes are unsaturated hydrocarbons, insoluble in water, and are olefinic compounds with molecular formulas that are multiples of isoprene (C5H8) (Table 3.). Based on their chemical structure, terpenes are categorized into hemiterpenes, monoterpenes, sesquiterpenes, diterpenes, triterpenes, and tetraterpenes.
Terpenoids | Analogue Terpenes | Number of Isoprene Unites | Number of Carbon Atoms | General Formula |
Hemiterpenoids | Isoprene | 1 | 5 | C5H8 |
Monoterpenoids | Monoterpenes | 2 | 10 | C10H16 |
Sesquiterpenoids | Sesquiterpenes | 3 | 15 | C15H24 |
Diterpenoids | Diterpenes | 4 | 20 | C20H32 |
Sesterterpenoids | Sesterterpenes | 5 | 25 | C25H40 |
Triterpenoids | Triterpenes | 6 | 30 | C30H48 |
Tetraterpenoids | Tetraterpenes | 8 | 40 | C40H64 |
Polyterpenoid | Polyterpenes | > 8 | > 40 | (C5H8)n |
The isoprene unit is a 5-carbon compound that forms the backbone of terpenes. Although it is considered the fundamental unit of terpenes, the synthesis of terpenes is not initiated by isoprene units. The isopentenyl diphosphate (IPP) unit and its isomer dimethylallyl diphosphate (DMAPP) are the biosynthetic precursors of terpenes [11].
IPP and DMAPP undergo condensation reactions catalyzed by geranyl pyrophosphate synthase (GPPS) and farnesyl pyrophosphate synthase (FPPS), producing GPP (C10_monoterpenes) and FPP (C15_sesquiterpenes). Geranylgeranyl pyrophosphate synthase (GGPPS) and farnesyl geranyl pyrophosphate synthase (FGPPS) catalyze similar enzymatic reactions to produce GGPP (C20_diterpenes) and FGPP (C25_sesterpenes). The terpene synthases superfamily (TPSs) further catalyzes the cyclization and rearrangement reactions of the GPP, FPP, GGPP, and FGPP precursors to form various terpene compounds [11].
Polarity
In chemistry, polarity refers to the uneven distribution of electric charge in a covalent bond or a covalent molecule. If the charge distribution is uneven, the bond or molecule is called polar; if it is even, it is called nonpolar. The polarity of a covalent bond arises because the two atoms forming the bond have different electronegativities. Bonds can fall into two extremes—polar and nonpolar. When the electronegativities of the ions forming the covalent bond are exactly the same, a completely nonpolar bond is formed. Conversely, when the difference in electronegativity between the two ions is so large that one ion completely takes an electron from the other, a polar bond (ionic bond) is formed (see Table 4).
Most terpenes are hydrocarbons, which means they are composed only of carbon and hydrogen atoms. They do not have highly electronegative atoms like oxygen or nitrogen to produce a significant dipole moment. Nonpolar terpenes (dipole moment close to 0 D) include monoterpenes such as limonene, pinene, myrcene, and isoprene, and the sesquiterpene farnesene. Slightly polar terpenes (1 D to 3 D) include alcohol terpenes such as linalool, menthol, and terpineol, and the ketone terpene borneol.
Polarity Type | Compounds |
Polarity | Campholenic Aldehyde |
Micro-polarity | 1,4-Cineole, α-Pinene Oxide, α-Terpineol, β-Caryophyllenol, β-Pinene Oxide, Borneol, Camphor, Caryophyllene oxide, δ-Terpineol, Dihydrocarveol, Dihydrocarvone, Dihydromyrcenol, Dihydroterpineol, Dihydroterpinyl Acetate, Estragole, γ-Terpinene, Geraniol, Iso Cyclemone E, Isoborneol, Isobornyl Acetate, Isolongifolanone, Isolongifolenone, Myrac aldehyde, Myrtenol, Perilla aldehyde, Pinocarveol, Terpinen-4-ol, Terpineol, Terpinyl Acetate, α-Terpinyl Acetate, δ-Terpineol |
Non-polarity | 3-Carene, α-Longipinene, α-Phellandrene, α-Pinene, α-Terpinene, β-Phellandrene, β-Pinene, Camphene, Dihydromyrcene, Dipentene, Isocitronellene, Isolongifolene, Isoterpinolene, Longifolene, Myrcene, Ocimene, p-Cymene, Pinane, Terpinolene |
Most monoterpenes and sesquiterpenes are non-polar or slightly polar. Generally speaking, non-polar compounds dissolve better in organic solvents/non-polar solvents. In terms of stability, non-polar compounds may be more stable under specific conditions, making them suitable for use in lubricants, insulating materials, and other inert applications. Conversely, non-polar compounds tend to have weaker mechanical properties or biological activities compared to polar compounds.
Improving non-polar or slightly polar compounds through addition reactions is a way to expand their applications. Terpene compounds are unsaturated hydrocarbons that contain C=C double bonds, which means they can undergo various addition reactions. In compounds with double or triple bonds, addition reactions open the multiple bonds, allowing the atoms on either side of the original bond to each connect with a new group. Addition reactions generally involve two molecules reacting to form one molecule, similar to combination reactions in inorganic chemistry. Common addition reactions include nucleophilic addition, electrophilic addition, free radical addition, cycloaddition, and polymerization addition.
Using monomer copolymers as an example, the two alkyl groups on the ring of β-pinene have always been considered to have only cationic polymerization activity, and the monomers capable of cationic polymerization are limited, resulting in a limited variety of β-pinene copolymers. Therefore, when terpene resins prepared from β-pinene are used in highly polar adhesives, due to drawbacks such as phase separation and weak interaction forces, they cannot achieve their optimal tackifying effect. If effective radical addition with a wide range of polar vinyl monomers, such as acrylonitrile and acrylic acid, can be achieved, it will diversify the copolymer products. The terpene resins after the addition reaction improve compatibility with polar adhesives. Additionally, the polar monomer structural units in the copolymer molecules contain polar groups, which can be used for macromolecular modification to obtain polymers with special functions. This will broaden the application of terpene-based polymers in composite materials.
On the other hand, polymerization addition can introduce crosslinkable, optically active β-pinene structural units into the polymer chains to modify polar monomer polymers. This can also improve the solubility of polar monomer polymers such as polyacrylonitrile and poly-N-substituted maleimide [12].
In 2023, the Chinese automaker SAIC-GM-Wuling Automobile Co., Ltd. applied to the China National Intellectual Property Administration for a “Composite Material and Its Preparation Method (CN117230577A) [13]”. According to the announcement, the automotive structural materials and comprises flax fibers and modified polypropylene fibers. The modified polypropylene fibers are polypropylene modified with isomerized terpene resin, which allows the modified polypropylene fibers and flax fibers to form a three-dimensional fiber network structure with triple action forces upon heating and compounding. Industry media have evaluated that this new composite material can improve performance while reducing carbon emissions.
Impact of biomass solvents on carbon footprint
In the electronics manufacturing industry, organic semiconductors have a lower carbon footprint than inorganic semiconductors. For example, organic solar cells (OSC), where the photovoltaic active layer is composed of carbon-based semiconductors. Using non-toxic raw materials, vacuum coating and solution processing can achieve cheap and fast coating of large areas [14]. According to relevant research, the lifecycle carbon footprint of OSCs is only 5–7 g CO2e kWh−1. In comparison, the lifecycle carbon footprint for the natural gas route is 400–500 g kWh−1, the coal route is 900–1000 g kWh−1, and the silicon-based semiconductor route is 50 g kWh−1[15] (Figure 4).
In the aforementioned study [14], Professor Daniel Corzo conducted computational research on Eucalyptol, d-limonene, β-Pinene, and L-Menthone as high-performance green inks for organic photovoltaics (OPV), OLEDs, and PFETs. He suggested that such a lifecycle can reduce the emission of halogenated solvents to remove carbon monoxide from the atmosphere and reuse carbon in the production stage of terpene compounds to synthesize OSCs. In his paper, the professor stated that carvone, linalool, anisole, and γ-valerolactone are future research directions.
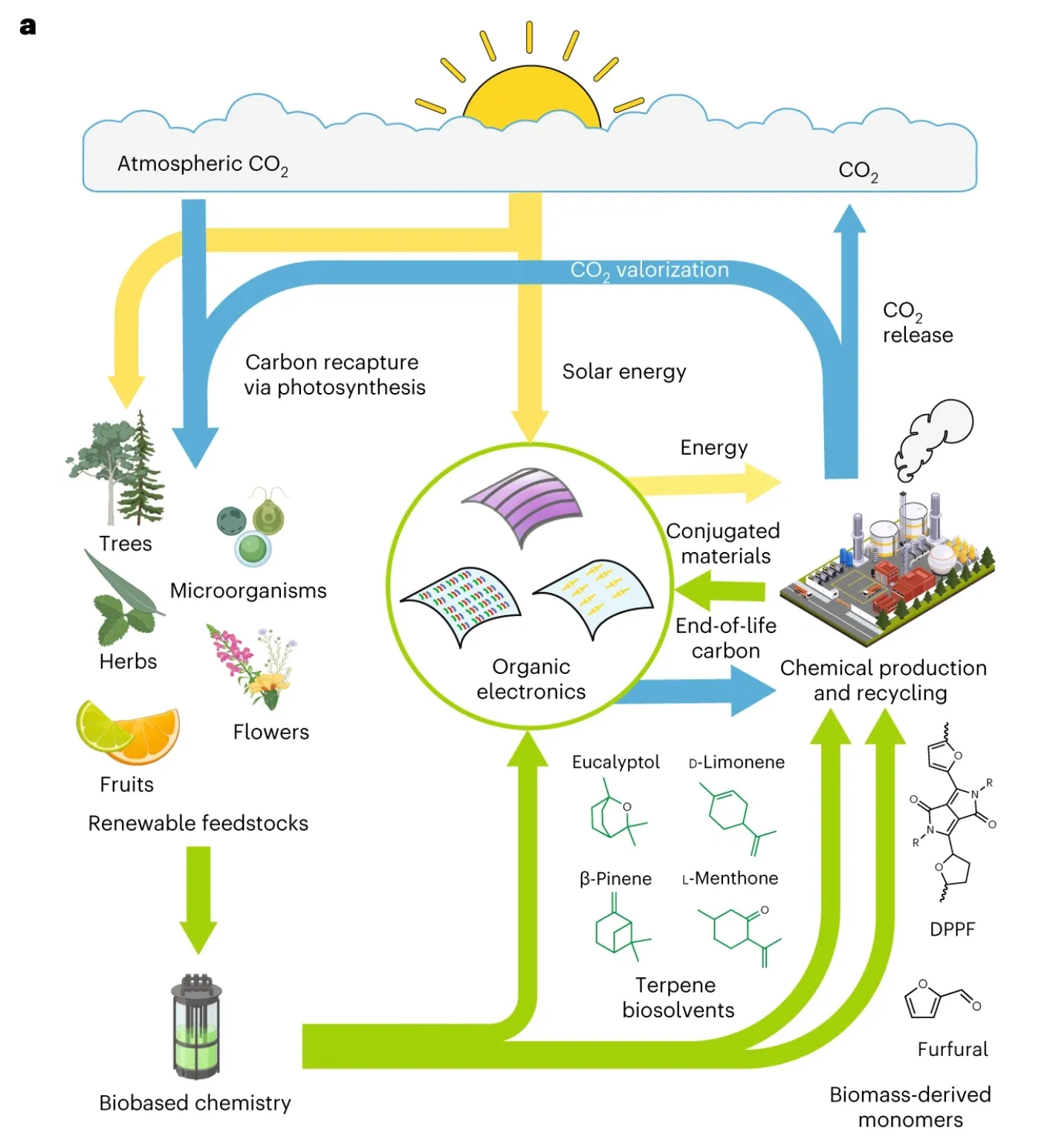
Figure 4. Toxicity and carbon footprint of solvents for OSCs. [Source: © Daniel Corzo al., High-performing organic electronics using terpene green solvents from renewable feedstocks, Nature Energy volume 8, pages62–73 (2023)]
References
- Kevin Chen al., Five questions on how biochemistry can combat climate change, BBA Advances, 2023
- Mirindi Eric Dusenge, Plant carbon metabolism and climate change: elevated CO2 and temperature impacts on photosynthesis, photorespiration and respiration, New Phytologist, 2019
- Jean-François MOROT-GAUDRY al., The path of carbon in photosynthesis, 2020
- Berkley J. Walker al., The Costs of Photorespiration to Food Production Now and in the Future, Annual Reviews, 2016
- Alex Guenther al., A global model of natural volatile organic compound emissions, JGR Atmospheres, 1995
- Guenther, A. B al., The Model of Emissions of Gases and Aerosols from Nature version 2.1 (MEGAN2.1): an extended and updated framework for modeling biogenic emissions, Geosci. Model Dev., 5, 1471–1492
- Greenhouse Gas and Energy Life Cycle Assessment of Pine Chemicals Derived From Crude Tall Oil and Their Substitutes, American Chemistry Council
- DONG JingXi al, Forest Resources and Estimated Resin Production of Pines in China – Based on the Data of Successive Nation-Wide Inventory of Forest Resources, Scientia Silvae Sinicae, 2009
- ZHU XIA al., Inheritance and Change of Chinese Rosin Production Technology and Its Social Support System, Journal of Dialectics of Nature, 2019
- The association’s exclusive interview with Peter Migchels, Global Adhesives Market Director at Kraton Corporation, CATIA, https://www.catia-china.com/post.html?id=614147b579ec7605b6021256
- Ninkuu V al., Biochemistry of Terpenes and Recent Advances in Plant Protection. International Journal of Molecular Sciences. 2021; 22(11):5710.
- LU Jiang al., Beta-pinene/polar vinyl monomer copolymer, and its preparing method and use, Patent CN1850874A
- GM Wuling Motors has applied for a patent for composite materials and their preparation methods, which can reduce the density of composite materials and carbon emissions. JRJ, https://finance.jrj.com.cn/2023/12/15212738787196.shtml
- Daniel Corzo al., High-performing organic electronics using terpene green solvents from renewable feedstocks, Nature Energy, 2022
- Moritz Riede al., Organic Solar Cells—The Path to Commercial Success, Advanced Energy Materials, 2020
Get the Quote
Contact our sales team to get the latest product specifications and product quotes.