Industry News, Coatings
Understanding the Function of Additives
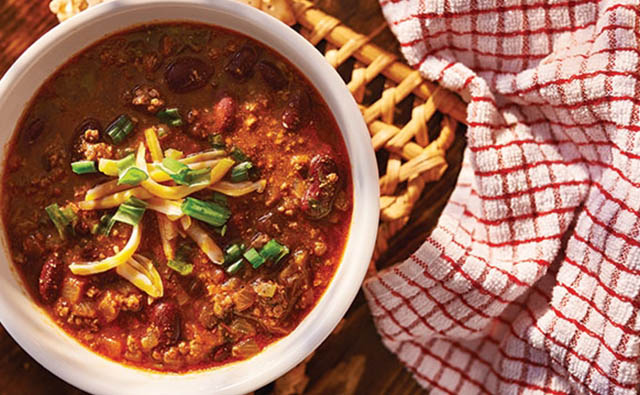
Industry News, Coatings
Why do we call them additives? They are added to a formulation and they add additional properties: what else could we call them? “How do they work” and “How do we design them”? These are more difficult questions.
Perhaps this is being overly simplistic, but a coating, at its heart, is a carrier or solvent and a film forming resin or binder. Actually egg tempera paint, which was used in pre-1400 medieval frescoes, was composed of egg yolk, pigment and water. Some artists today still use egg tempera. One could also say that chili is meat, beans and ketchup. While partially true, there are countless chili recipes with a multitude of spices, different meats, and many more sometimes secret ingredients (ask anyone from Texas!). By analogy, additives are the flavors and spices of a coating.
Modern coatings are complex formulas with thickeners, emulsifiers, dispersants, pigments, defoamers, leveling agents, photo-stabilizers, curing catalysts and other additives. These additives enhance the inherent properties of the resin.
Flow and leveling agents are probably the most important class of additives. A myriad of defects can occur in the forming film with colorful names like fisheyes and orange peel, and the very technical sounding Bénard cells.1 A critical parameter in the formation of these defects is localized surface tension gradients which physically form the defect and hold it while the film dries. Surfactant-based flow and leveling agents work by reducing the surface tension of the carrier liquid, which allows the liquid to flow more easily and reduces the strength of the gradients.
Additive designers, a self-imposed and admittedly lofty term, have several base chemistries to choose from when building surface tension reducing agents. In general, amphiphilic molecules, derived from hydrocarbon-based hydrophobic groups, can lower aqueous surface tension from pure water’s 72 mN/m into the 30 mN/m range. Polydimethylsiloxane (aka silicone) hydrophobe-based materials lower surface tension into the 20 mN/m range and perfluoroalkyl surfactants into the 15-20 mN/m range. There are some notable exceptions in the organic materials such as acetylenic diols and small linear alcohols that can reach into the high 20 mN/m range.2,3
The use level needed for a surfactant also changes for the different basic chemistries. In water, surfactants have a measurable property called the critical micelle concentration.4 This is the concentration at which micelles are formed and where surface tension reduction is greatest. For our purposes this is essentially the minimum effective use level. Organic surfactants typically have a CMC in the 10-2 range, silicone surfactants in the 10-3 range and fluoroalkyls can be even lower. Hence the typical use levels of 0.1%, which is well above these CMCs, are effective.
Gloss improvement from additives is generally a result of improved flow and leveling. An even, defect-free coating has a high distinctness of image (DOI)5 allowing one to see the gloss of the resin to its maximum effect. Additive designers can also use flow and leveling agents with higher refractive indices which will give a higher gloss.
The lower the surface tension, the more powerful the flow and leveling agent is. However, with great power sometimes comes great problems – to borrow a phrase from Spiderman. Surface active agents are driven to all of the interfaces including gas/liquid, liquid/solid, etc. This can cause many problems for formulators.
From the perspective of a coating being applied, surface active agents at the liquid/solid interface can cause poor adhesion to a substrate. The same phenomenon at the cured film/air interface, can provide a surface that is difficult to wet from the perspective of the next coat.
In order for a liquid to be able to wet a surface the surface tension of the liquid must be lower than the surface energy of the surface. Otherwise the liquid beads up as pure water beads on most surfaces due to its inherently high surface tension. If the surface which needs to be wetted is a previous coating, the surfactants on what is now the cured solid/air interface of the previous coat will lower the surface energy. This leads to wetting and overcoatability concerns.
One solution to overcoatability is to add a surfactant to the next liquid coating. This would be acting as a wetting agent by lowering the surface tension of the liquid in order to wet out the dried previous coat. Wetting agents and flow and leveling agents are similar chemical answers and differ primarily in the perspective or the problem being solved. This is why those terms are often intermingled.
At the air/liquid interface, low-surface-tension agents stabilize foam more efficiently than high-surface-tension materials. This is yet another interfacial problem. Again the solution, a defoamer in this case, needs to have a lower surface tension than the foam stabilizer to be effective. With all of these interfaces, is it any wonder the use of additives is so complex?
Sometimes one wants to create a coating that is not able to be wetted, for example an anti-grafitti, anti-fouling, or release coating. In that event, migration of these surface-active agents to the interfaces helps. Additive designers would favor silicone or fluoroalkyl surfactants for these applications which can give both flow and leveling, wetting and release.
However, the most potent of these release agents are not the surfactants. A minimally modified or unmodified silicone polymer, fluorocarbon or even hydrocarbon is the most efficient way to accomplish this surface effect. While surfactants are designed to be miscible with the carrier and binders, this diminishes both the amount and the potency of the low-surface-energy materials at the interface. An immiscible polymer will migrate very quickly to the interface and cause maximum release properties.
We all learned that oil and water are not miscible, and of course water with silicone oil and water with fluorocarbon oils do not mix either. But some are at first surprised that silicone, fluorocarbon and hydrocarbon oils also do not mix with each other. The additive designer relies on this immiscibility and designs release additives that are chemically closer to pure silicon or fluorocarbon because these are the most potent release agents. The art of this balancing act is to add enough chemical modification to prevent defects and, thereby, send us back into a loop looking for flow and leveling.
Importantly since these materials are not reacted into the matrix, they are free to migrate through the cured coating to the interfaces. The expression “blooming silicone” is not an Australian condemnation, but rather an accurate description of how these work. They continue to migrate or bloom to the interfaces and further lower coefficient of friction (COF), surface energy and tape release properties.
The UV-cured overprint varnish industry has found a solution to this migration. These formulators commonly use acrylate functional silicones which migrate in the liquid coating to give the slip and release properties desired, but then react into the matrix upon curing to prevent blooming.6 The same technique can be used with other cure mechanisms if the additives are suitably functionalized to react into the matrix. Care should be taken in slower-cure systems as migration may take days to stop as the cure continues.
Mar and stain resistance are also improved by these low-surface-energy materials at the solid/air interface of the cured coating.7 Mar resistance is a consequence of the slipperiness of the surface; materials that would damage the coating tend to slide across it instead. The coating is not actually harder, but the mar resistance is improved without changing the crosslink density of the film.
Mar and abrasion resistance improvement can also be obtained with increased crosslinking from additives such as organo-functional tri-alkoxy silanes. These are designed to react into the matrix with multiple crosslink sites, a concept that also works with high-functional reactive polymers.8
Stain resistance improvement is due to the low surface energy and poor miscibility of these agents at the interface. Although this property is very dependent on the stain, fluoroalkyl additives are often better for this since less stains are miscible with those.9
It is important how these additives are aligned at the interface, especially for stain resistance. Different molecular architectures lay at the interface differently and can give very different performance.10
We touched on foam in the above discussion of flow and leveling agents. Surfactants, including flow and leveling agents and emulsifiers, migrate to the air/liquid interface formed upon mechanical agitation of the coating and some of them stabilize the foam. A surface active agent that does this is referred to as a profoamer. Foam is a property not often desired in coatings.
A defoamer is a material that will cause foam to break when added to it, the simplest way of demonstrating this is to add alcohol to a stable foam – the foam breaks. This low-surface-tension, low-viscosity molecule displaces the profoamer molecules at the interface and allows for a quick collapse of the foam. (As an aside, this is why you add tequila before blending a frozen margarita.
Alcohol is a defoamer, but not an antifoam.) In coatings, many mineral-oil-based foam control agents provide this defoaming property.
An antifoam is a more complex additive; it prevents the formation of foam during the agitation. Antifoams work by migrating to the interface, spreading across it and spanning the air gap to rupture the interface.11 Additive designers develop antifoams which nearly all have an immiscible, low-surface-energy oil and an oil-soluble mechanical particle to accomplish this feat.
Silicone-based antifoams are the norm in coatings and can be used down to ppm levels in many cases. An important but not well known fact is that antifoams can get used up. In an application with constant agitation like a laundry machine, the antifoam eventually gets mechanically dispersed to where it is no longer effective.
Degassing or microfoam elimination is yet another problem. The viscosity and flow resistance of modern carriers, i.e., waterborne and high solids solvents, prevent the smallest of the foam bubbles formed from migrating and coalescing. Buoyancy of a gas bubble in a liquid is proportional to size, so the smallest bubbles do not have enough force to bring them into macrofoam. The result, after curing, is pinhole formation and gas pockets in the coating.
The designer’s solution to microfoam is to go back to surface active materials choosing those which will quickly migrate to these interfaces and affect the stability and size of these tiny bubbles. Cloud point defoaming, which uses surface active materials with low solubilities, or cloud points, are a common mechanism here.12 Foam control theory is established far beyond what we are able to present in the scope of this brief review. Readers are encouraged to see references cited herein.
Hopefully you have a better understanding of the complexity and power of additives. In my career, the most common philosophical question I have heard is ‘why can’t we design one additive to do it all’? This additive would lower surface tension to eliminate defects, but not so much as to cause intercoat adhesion or prevent overcoat problems (except in anti-graffiti and marine coatings where we want overcoat problems – but still need adhesion). Also it would give mar and stain resistance, high gloss but not too high, and no foam. The answer is – we’re working on it.
1 Pierce, P.E.; Schoff, C.K. Coating Film Defects, Rev. Ed. 1994, Federation of Societies for Coatings Technology, Blue Bell, PA.
2 Ferri, J.K.; Stebe, K.J. Which surfactants reduce surface tension faster? A scaling argument for diffusion-controlled adsorption. Advances in Colloid and Interface Science 2000, 2, 85(1), 61-97.
3 Zhang,P. et.al. U.S. 7,129,199 B2, 2006.
4 Ruckenstein et.al. J. Phys. Chem. 1975, 79 (24), 2622–2626.
5 ASTM D5767.
6 Ruckle, R.E.; Marengo, P.A. The Effect of Silicone Additive Structure on Foaming in Radcure Systems. In Proceedings of the Radtech Symposium, 1994, Radtech NA.
7 Ruckle, R.E.; Cheung, T.; Horne, A. Incorporation of Reactive Silicones into Various Coatings Films and the Resulting Properties. Presented at the Western Coatings Symposium, 2013, Los Angeles Chapter of the Society of Coatings Technology (LASCT).
8 Ruckle, R.E.; Cheung, T. A Structure Property Study of Epoxy Resins Reacted with Epoxy Silicones. Proceedings of Sampetech, 2014, Society for the Advancement of Material and Process Engineering.
9 Ruckle, R.E.; Cheung, T. Incorporation of Fluoro-Silicones in Coatings Films and the Resulting Properties. In Proceedings of the Waterborne Symposium, 2014, University of Southern Mississippi.
10 Ruckle, R.E.; Cheung, T. Properties of Silicone Modified UV Cured Acrylate and Epoxy Coatings Films. In Proceedings of the Waterborne Symposium, 2013, University of Southern Mississippi.
11 Koczo, K. et.al., J. Colloid and Interface Science 1994, 166, 225-298, (and associated references).
12 Nemeth et.al., J. Colloid and Interface Science 1998, 207, 386-394, (and associated references).